PI: Grace Y. Hsuan, Dept. of Civil, Architectural & Environmental Engineering
Co-PI: Richard Cairncross, Dept. of Chemical and Biological Engineering
Co-PI: Christopher Li, Dept. of Materials Science and Engineering
In the last three decades, Polyethylene (PE) has become a widely used material in many of the civil engineering products, including various types of geosynthetics, pressure and corrugated pipe, and retention tanks. While products made from PE are lightweight, easy to install, and resist many chemicals, their mechanical and chemical properties can be significantly enhanced by adding a small amount of nanoclay, making PE/clay nanocomposites an attractive new class of engineering materials.
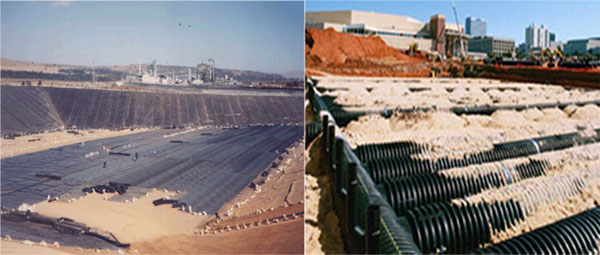
Figure 1: Civil Engineering Applications of PE pipes
In order to adopt nanocomposite as a civil engineering material, it must possesses the same service life of the system, which is generally expected to last from 50 for transportation applications and over hundred years for environmental applications. Despite a large amount of research on material properties and processing techniques of PE nanocomposites, the long-time performance is still poorly understood. Long-term degradation of PE properties is primarily due to environmental oxidative degradation.
PE/Clay Nanocomposite
Mechanical properties of PE can be significantly improved by adding clay and carefully processing it to form nanocomposites
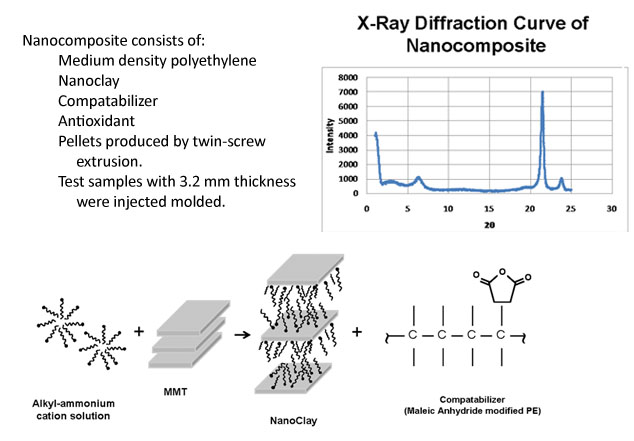
Figure 2: Composition of nanocomposite
The transition metal ions, organic modifiers and compatibilizers enhance compatibility between clay and PE, but they also have a negative effect on processing and durability of PE articles. They are less stable under high temperature conditions, and can promote generation of free radicals.
Antioxidants (AO) are essential in prolonging the service life of polyethylene products. During aging, AO degrades preferentially to PE, so once the AO is depleted, the material is no longer protected from environmental oxygen. Depletion of AO from neat PE had been studied in detail, but research on AO depletion in PE-clay nanocomposites is minimal. Our research focusses on experimental evaluation of AO depletion and mathematical modeling to represent the mechanism of AO depletion.
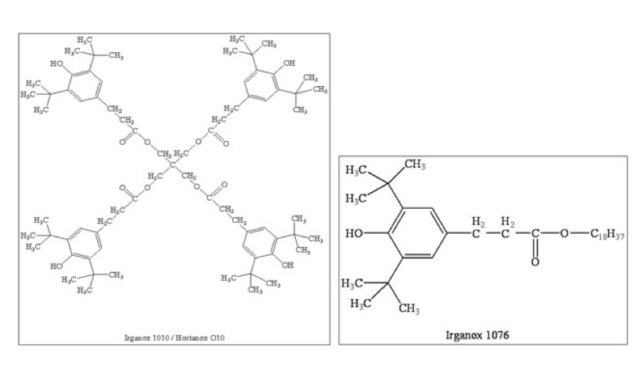
Figure 3: Chemical Structure of (a) Irganox®1010 (b) Irganox®1076
Test Materials, Aging Condition, and Method
Consumption of AO in PE under normal atmospheric conditions may take several decades. Therefore, accelerated thermal aging was used in this study. Samples were held at 85°C in a circulated air atmosphere. Data spanning over two years have been collected so far.
Collaborators on this research project have conducted experiments to monitor AO depletion for more than a year using 'Oxidation Induction Time' (OIT) test method. OIT is an indirect measure of active AO concentration present in a polymeric sample. OIT and AO concentration are expected to be linearly proportional in case of the selected AOs (Irganox®1010, Irganox®1010, Irgafos®168) in PE matrix.
Table 1: Composition of nanocomposites samples
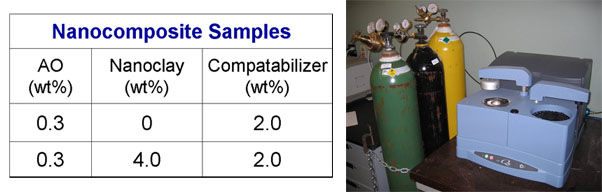
Figure 4: DSC apparatus used to run OIT tests
Results
Experimentally determined OIT profiles are shown in figure 2 (a) and (b) for neat PE and PE-clay nanocomposites respectively with Irganox®1010
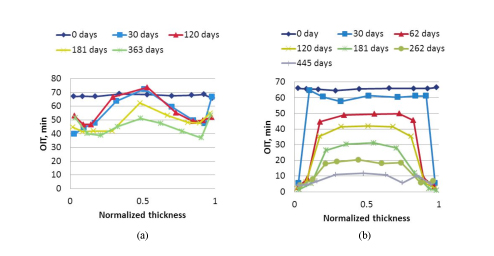
Figure 5: (a) OIT profile of Neat PE at different aging times (b) OIT profile of PE-Clay nanocomposite at different aging times
The primary cause of AO depletion is by reaction with peroxide free radicals. In the presence of oxygen, alkyl free radicals on polyethylene react to form peroxide free radicals, which if not consumed by AO will lead to a chain of further free radical reactions. Thus, in a thick polymer, AO consumption will largely depend on diffusion of oxygen in the sample.
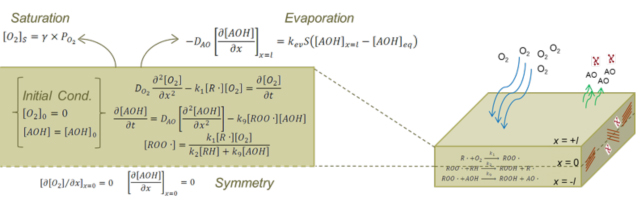
Figure 6: Model to describe AO depletion
The oxygen concentration can be solution of the following diffusion and reaction equation:
where, D_(O_2 ) is effective oxygen diffusivity, [R∙] is the alkyl free radical concentration, and k_1 is the effective rate constant for reaction of O_2 with R∙ to form peroxide free radical. The initial oxygen concentration inside the sample is assumed to be negligible. The oxygen concentration on the sample surface is assumed to be the product of the partial oxygen pressure around the sample and the coefficient of solubility. At the center of a sample with symmetric boundary conditions are applied.
The overall mass balance on AO produces the following diffusion-reaction equation:
where D_AO is the effective diffusivity of AO in PE/Clay nanocomposite samples, [AOH] is the AO concentration, [ROO∙] is peroxide free radical concentration, and k_9 is the effective rate constant. The initial AO concentration is assumed to be uniform throughout the sample thickness. The flux of AO at the center of sample is zero due to symmetry. AO evaporation through surface is assumed.
Table 2: Models to describe AO degradation
Finite difference method was used to convert the PDEs into a set of ODEs which were then solved simultaneously using a stiff ODE solver in MATLAB.
The model contains many parameters that are extracted from various reported literature. Some of the parameters were adjusted to achieve qualitative agreement between model predictions and experimentally-measured AO profiles.
Table 3: Simulations from the models
Summary
- Three models of PE/AO diffusion & reaction created that qualitatively match features of experimental OIT profiles:
- Model I has constant alkyl free radical
- Model II has no generation of alkyl free radicals and could lead to residual free radicals after AOs consumed.
- Model III has both generation and consumption of alkyl free radical
- To achieve good model-experimental agreement:
- Higher initial alkyl free radicals required in Nanocomposites than in neat PE
- Uniform oxygen necessary for flat AO profile in core
- Evaporation needed for depleted surface zone
Acknowledgements
Polyethylene resin was supplied by Chevron-Phillips Chemical Company.
Nanocomposite samples were prepared using facility in the Nanocor R & D lab.
Iftekhar Ahmad and Shan Cheng are the graduate students working on this phase of the project.